- Meaning of Structure and Bond.
- Nature of the Chemical Bond.
- Types of Bonds.
- Types of Bonds Across a Period.
- Summary: Characteristics of Bonds
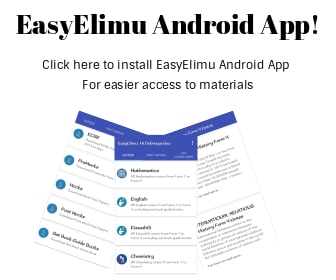
Meaning of Structure and Bond.
Bond:
- The mutual force of attraction that holds particles together when atoms (similar or different) combine during chemical reactions.
Structure:
- A regular pattern of particles in a substance held together by chemical bonds.
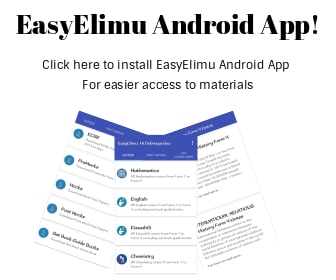
Nature of the Chemical Bond.
- Atoms are made up of energy levels and nucleus.
- The energy levels contain electrons while the nucleus contains protons and neutrons.
- The electrons are negatively charged, protons are positively charged while neutrons are electrically neutral.
- The noble gases are chemically inert since their outermost energy levels are completely filled with the maximum possible number of electrons.
- Thus noble gases have either a stable duplet state (2) like in helium or a stable octet configuration (2.8 or 2.8.8) as in neon and argon respectively.
- Other atoms are unstable because the outermost energy levels are not yet completely filled with the maximum possible number of electrons.
- To attain the stable duplet or octet noble gas configuration, such atoms lose, gain or share their valence electrons.
- It is the act of losing, gaining or sharing valence electrons that lead to the chemical bonds.
- When atoms gain or lose valence electron(s) they become charged forming anions and cations respectively.
- Particles of the same charge repel each other while particles of different charges attract one another.
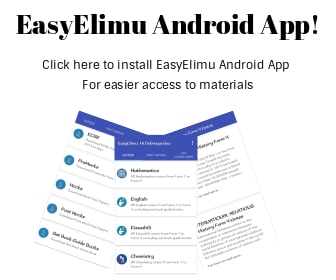
Types of Bonds.
- There are three main types of chemical bonds:
- Ionic bonds
- Covalent bond
- Metallic bond
Ionic Bonds/Electrovalent Bond.
- Is a bond formed due to complete transfer of electrons from one atom to another resulting into two oppositely charged ions.
Formation of an Ionic Bond.
- Formed due to complete transfer of electrons from one atom to another; and mainly formed between a metal and a non-metal.
- This occurs in a bid for both atoms to acquire a stable noble gas configuration.
- One atom loses all its valence electrons thus forming a cation (positively charged ions).
- The other atom gains all the lost valence electrons forming an anion (negatively charged ion).
- The cation and the anion are oppositely charged and thus develop a mutual force of attraction between them which is the ionic / electrovalent bond.
Illustration: formation of ionic bond between sodium and chlorine to form sodium chloride.
- Sodium metal (atomic number 11) has electronic configuration 2.8.1 and thus unstable with 1 valence electron.
- Chlorine gas (atomic number 17) has electronic configuration 2.8.7 and thus unstable with 7 valence electrons.
- Sodium is more electropositive (the tendency to lose electrons to form cations) than chlorine while chlorine is more electronegative (the tendency to gain electrons to form anions).
- Sodium loses its single valance electron to form sodium ion with electronic configuration 2.8 and a net charge of +1 (Na+)
- Chlorine atom accepts the single electron lost by sodium to form a chloride ion with electronic configuration 2.8.8 with a net charge of -1 (Cl-).
- The positively charged sodium ion and the negatively charged chloride ion attract each other.
- The electrostatic forces of attraction develop between the two oppositely charged ions and this constitutes the ionic bond.
- Compounds formed due to ionic bonding are thus called ionic compounds.
Diagrammatically:
Examples of ionic compounds.
- Sodium chloride.
- Potassium fluoride.
- Magnesium oxide
- Aluminium (III) oxide.
Giant Ionic Structures
- Ionic bonding results into one type of structure, the giant ionic structure.
- This is a type of structure in which all ions are bonded with strong ionic bonds throughout the structure.
- Each ion in the giant ionic structure is surrounded by several others resulting into giant pattern of several ions, hence giant ionic structure.
- Most ionic substances with the giant ionic structure are crystalline in nature, made up crystals.
- A crystal is a solid form of a substance in which the particles are arranged in a definite pattern repeated regularly in 3 dimensions.
Illustration of the giant ionic structure: sodium chloride structure.
- The NaCl structure consists of many Na+ and Cl- arranged and packed in a regular pattern.
- Each Na+ is surrounded by six Cl- that are equidistant from it.
- Similarly each Cl- is surrounded by six Na+ that are equidistant from it.
- This pattern occurs repeatedly in all directions.
- The result is a giant of ions in all directions hence giant ionic structure.
Diagram of the cubic structure of sodium chloride.
Properties of Giant ionic Structures.
They are hard and brittle.
- Ionic solids are hard because each ion is held in the crystal by strong attractions from the oppositely charged ions around it.
- They are brittle and thus may be split cleanly (cleaved) using a sharp-edged razor.
- When a crystal is tapped sharply along a particular plane it is possible to displace one layer of ions relative to the next.
- Due to the displacement, ions of similar charge come together leading to repulsive forces between the portions of the crystals.
- This forces the two portions of the crystals to split apart.
Diagram: crystal cleavage in ionic compounds.
They have high melting and boiling points.
- They have strong electrostatic forces / ionic bonds / electrovalent bonds between the oppositely charged ions throughout the structure which require large amounts of energy to break.
Solubility.
They are soluble in polar solvents like water, ethanol and acetone (propanone)
- Water contains highly polar molecules.
- The positive ends of the polar water molecules are attracted to the negative ions in the crystal, and the negative ends of the water molecules are attracted to the positive ions in the crystal.
- This results to the formation of ion-solvent bonds which leads to release of energy.
- This energy is sufficient to cause the detachment of ions from the crystal lattice hence dissolution.
- This detachment of ions is called solvation , and the energy required for this is called solvation energy.
- Where the solvent is water the ions are said to have been hydrated , and the energy involved in the process is called hydration energy.
Diagrams: hydrated positive and negative ions.
They are insoluble in non-polar organic solvents like tetrachloromethane, benzene and hexane.
- Non-polar molecules are held together by weak intermolecular forces, the Van der Waals forces.
- The Van der Waals are much smaller in magnitude compared to the ionic bonds in the ionic solid crystal lattice.
- Thus the ion-ion interactions in the ionic solid are stronger than the solvent-solvent interactions in the solvent or the solvent-ion interactions between the solid and the solvent.
- Thus the non-polar solvent molecules cannot penetrate the ionic lattice to cause salvation.
Electrical conductivity.
- Ionic substances do not conduct electric current in solid state.
- The ions are held in static positions in the solid crystal lattice and thus cannot move to conduct electric current.
- They conduct electric current in molten and solution (aqueous) states.
- In molten and aqueous states the ions are free and mobile and thus move about conducting electric current.
Gradation in properties of some ionic compounds of sodium.
Property of compound | Compound of sodium | ||||
Sodium fluoride | Sodium chloride | Sodium bromide | Sodium iodide | ||
Solubility in water | Soluble | Soluble | Soluble | Soluble | |
Melting point (oC) | 993 | 801 | 747 | 661 | |
Boiling point (oC) | 1695 | 1413 | 1390 | 1304 | |
Electrical conductivity | Solid | Does not | Does not | Does not | Does not |
Molten / solution | Conducts | Conducts | Conducts | Conducts |
- Solubility of the compounds decrease from sodium fluoride to sodium iodide.
- Melting and boiling points decrease from sodium fluoride to sodium iodide.
The Covalent Bond.
- Refers to a bond formed when two atoms of the same or of different elements share electrons to become stable.
- Formation of covalent bond between atoms (similar or dissimilar) result to the formation of a molecule.
- Covalent bonds are usually formed by the association of non-metals.
- A molecule is a group of atoms (two or more) of the same or different elements that are held together by strong covalent bonds.
Formation of a Covalent Bond.
- Covalent bonding is brought about by the facts that the electro-positivity and the electro-negativity of the elements involved are very close.
- For that reason, none of the atoms can completely lose its valence electrons to the next atom.
- For this reason, both atoms donate electrons which are then shared between them.
- Both atoms thus attain a stable noble gas (duplet or octet) configuration.
Illustrations:
Formation of Chlorine Molecule.
- Each chloride atom has electronic configuration 2.8.7 and thus need to gain a single electron in the outermost energy level to attain a stable noble gas configuration.
- Since both chloride atoms have same electro-negativities, none will easily lose an electron to the other.
- For this reason, both donate the number of electrons required by the other atom (in this case 1), which they share between them.
- Thus chlorine molecule is formed by sharing 2 electrons between two chlorine atoms, hence a single covalent bond.
Diagram: formation of chlorine molecule.
Formation of oxygen molecule.
- Each oxygen atom has electronic configuration 2.8.6 and thus need to gain 2 electrons into the outermost energy level to attain a stable noble gas configuration.
- Since both oxygen atoms have same electro-negativities, none will easily lose an electron to the other.
- For this reason, both donate the number of electrons required by the other atom (in this case 2), which they share between them.
- Thus oxygen molecule is formed by sharing 4 electrons (2 from each atom) between two oxygen atoms, hence a double covalent bond .
Diagram: the oxygen molecule.
- A single covalent bond is represented in dot (.) and cross (x) diagrams using two dots, two crosses, a dot and a cross or a single line ( - ) between the atoms involved in the bond.
- Thus a single covalent bond like in chlorine can be represented as Cl - Cl, a double covalent bond like in oxygen can be represented as O = O while a triple covalent bond like in nitrogen can be represented as N ≡ N.
Exercise: Draw the Dot (.) and cross (x) diagrams for the following covalent compounds.
- Hydrogen, H2
- Hydrogen chloride, HCl
- Nitrogen, N2
- Water
- Carbon (IV) oxide, CO2
- Ammonia gas, NH3
- Phosphene, PH3
- Methane, CH4
- Ethane. CH2CH2
- Ethyne, C2H2
- Ethanol, C2H5OH
- Bromoethane, C2H4Br.
The Coordinate Bond.
- Refers to a type of covalent bond in which the shared pair of electrons forming the bond is contributed by only one of the atoms forming the bond.
- It is also called the dative bond.
Formation of ammonium ion.
- Occurs when an ammonia gas molecule combines with a hydrogen ion (proton).
- All the atoms in the ammonia molecule have a stable noble gas configuration and thus the molecule is stable.
- However the nitrogen in the ammonia molecule has a lone pair of electrons (electrons that have not yet been used in bond formation)
- The hydrogen ion has lost its outermost single valence electron to form the hydrogen ion.
- Thus the hydrogen ion has no electron(s) in its outermost energy level.
- To be stable the hydrogen needs two electrons in its outermost energy level.
- The hydrogen ion thus accepts bonds with the lone pair (2) of electrons in the nitrogen of the ammonia molecule forming a dative bond.
- The total number of electrons in the ammonium ion is 11 while the total number of electrons is 10 leading to a net positive charge of +1
- In a dot (.) and cross (x) diagram where the covalent bond is represented by horizontal lines ( ), the dative / coordinate bond is represented by an arrow (→) pointing the atom that “accepts” the electrons.
Diagram: formation of ammonium ion.
Formation of hydroxonium ion (H3O+)
- Occurs when a water molecule combines with a hydrogen ion (proton).
- All the atoms in the water molecule have a stable noble gas configuration and thus the molecule is stable.
- However the oxygen in the ammonia molecule has 2 lone pairs of electrons // four electrons (electrons that have not yet been used in bond formation)
- The hydrogen ion has lost its outermost single valence electron to form the hydrogen ion.
- Thus the hydrogen ion has no electron(s) in its outermost energy level.
- To be stable the hydrogen needs two electrons in its outermost energy level.
- The hydrogen ion thus accepts and bonds with two of the four electrons in the oxygen of the water molecule forming a dative bond / coordinate bond.
- The total number of electrons in the hydroxonium ion is 11 while the total number of electrons is 10 leading to a net positive charge of +1
Diagram: formation of the hydroxonium ion.
- The hydroxonium ion (H3O+) can still further react with another hydrogen ion to form another ion of the formula H4O2+ .
- This is due to the presence of a single lone pair of electrons in the structure of the hydroxonium ion (H3O+).
- The H4O2+ cannot however react further since all the valence electrons in all its atoms have been used in bonding leaving no lone pairs.
Further examples of dative covalent bonds in other compounds.
- Carbon (IV) oxide
- PH4+
- Aluminium chlorine dimer (Al2Cl6).
- Ammonia-aluminium chloride vapours complex, AlCl3 .NH3
- Substances with covalent bonds form two main types of structures:
- Molecular structures
- Giant atomic // Giant covalent structures.
The Molecular Structures.
- Refers to a structure in which covalent bonds holds atoms together to form molecules and the resultant molecules are held together by intermolecular forces.
- Substance with molecular structures are usually gases or liquids at room temperature.
- Few solids such as sulphur, iodine, fats, sugar, naphthalene and paraffin wax also have molecular structures.
- The intermolecular forces in molecular structures are of two types:
- Van der Waals
- Hydrogen bonds
The Van der Waals.
- Are the weakest form of intermolecular forces due to induced dipole attractions between molecules.
- As the size of the molecule increases, the number of constituent electrons increases leading to increase in strength of the induced dipole induced dipole interactions.
- The strength of the Van der Waals thus increases as the molecular size increases.
- This explains the trend in boiling points for the halogens, which increase from fluorine to iodine.
- From fluorine to iodine the size of the atoms and hence the molecules increases leading to increase in molecular masses that lead to stronger induced dipole induced dipole interactions hence increasing strength of the Van der Waals (from F2 to I2 )
- This also applies for the increase in boiling points for the homologous series of alkanes.
Diagram: Illustration of Van der Waals forces
- Iodine
- Graphite.
The hydrogen bonds.
- Is an intermolecular force in which the electropositive hydrogen atom of one molecule is attracted to an electronegative atom of another molecule.
- The essential requirements for the formation of a hydrogen bond (H bond) are:
- A hydrogen atom attached to a highly electronegative atom.
- An unshared pair of electrons on the electronegative atom.
- This explains why hydrogen bonds are common in molecules in which hydrogen are bonded to highly electronegative atoms like nitrogen, oxygen and fluorine.
The formation of hydrogen bonds
- Occurs when hydrogen atom is bonded to a highly electronegative atom like nitrogen, oxygen and fluorine.
- The electrons in the covalent bond (between hydrogen and the more electronegative atom) are drawn towards the electronegative atom.
- Hydrogen atom has no electrons other than the one it contributes to the covalent bond, which is also being pulled away from it (by the more electronegative atom).
- Hydrogen atom has no outer energy level of electrons making the single proton in the nucleus unusually bare.
- The proton is thus readily available for any form of dipole-dipole attractions.
- The bare proton of the hydrogen atom thus attracts the more electronegative atom (e.g. N, O and F) on either side.
- It thus exerts an attractive force on the more electronegative atom hence bonding them together.
- The two (more electronegative) larger atoms are drawn closer with a hydrogen atom effectively buried in their electron clouds.
- This constitutes the Hydrogen bond.
Illustration: formation of hydrogen bonds in water.
- In water two hydrogen atoms are bonded to an oxygen atom which highly electronegative.
- The electrons in the covalent bond (between each hydrogen and oxygen atom) are drawn towards the more electronegative oxygen atom.
- The hydrogen atoms have no electrons other than their share of those in the covalent bond, which are also being pulled away from them by the oxygen atom.
- Hydrogen atoms have no outer energy level of electrons making the single proton in their nucleus unusually bare.
- The proton is thus readily available for any form of dipole-dipole attractions (with oxygen in this case).
- The bare proton of each of the hydrogen atoms in one molecule thus attracts the more electronegative oxygen atom of the neighbouring molecule.
- Each hydrogen atom thus exerts an attractive force on the oxygen atom of the next molecule hence bonding them together.
- The electronegative oxygen atom of one molecule is drawn to the electropositive hydrogen atom of the next molecule with the hydrogen atoms effectively buried in the electron clouds of oxygen.
- This constitutes the Hydrogen bond in the water molecule.
Diagram: hydrogen bonds in water molecules.
- Other compounds with hydrogen bonds include ethanol, ammonia, hydrogen fluoride etc.
- Hydrogen bonds are much stronger than the weak Van der Waals forces but still weaker than the covalent bonds.
Effect of hydrogen bonding on properties of molecular substances.
- Hydrogen bonding tends to disrupt the gradation in physical properties of molecular substances in relation to molecular weights.
- The effect on molecular masses on the melting and boiling points only apply when the intermolecular force is the same.
Examples.
- Both ethanol (C2H5OH) and dimethyl ether (C2H6O) have the same relative molecular mass of 46. However the boiling point of ethanol is higher at 78.5oC than that of dimethyl ether at only -24oC since even though both have molecular structures with covalent bonds between the atoms, the intermolecular forces in ethanol are hydrogen bonds which are much stronger than the intermolecular forces in dimethyl ether which are weak Van der Waals forces.
- Ethanol (C2H5OH) has a molecular mass of 46 while butane (C4H10) has a molecular mass of 58, yet the boiling point of ethanol is higher than that of butane since both have molecular structures with covalent bonds between the atoms. However, the intermolecular forces in ethanol are hydrogen bonds which are much stronger (and require more energy to break) than
the intermolecular forces in dimethyl ether which are weak Van der Waals forces. - Molecular substances are generally insoluble in polar solvents like water. However those with hydrogen bonding as the intermolecular forces are soluble in water since the hydrogen bonding confers them some polarity. Examples: Sugar, ethanol, ethanoic acid etc
Properties of molecular structures.
Melting and boiling points.
- The generally have low melting and boiling points. Although the atoms forming the molecules are held together by strong covalent bonds, the
intermolecular forces are weak Van der Waals forces which require low amounts of energy to break.
Heat and electrical conductivity
- They are poor conductors of heat and electricity at any state since they have neither delocalized electrons nor free mobile ions for electrical conductivity.
Solubility
- Molecular substances are generally insoluble in polar solvents like water but soluble in non-polar organic solvents like benzene because in polar solvents like water there are strong water water attractions which are considerable stronger than the intermolecular forces (Van der Waals) attractions or molecule water (solvent) attractions, making the molecules unable to penetrate the water (solvent) structure for dissolution to occur.
- In non-polar solvents like benzene, the benzene-benzene attractions are similar in strength to the intermolecular forces or the molecule benzene (solvent) attractions, enabling the molecules to penetrate the solvent thus allowing dissolution.
- Molecular substances with hydrogen bonds as the intermolecular forces are soluble in polar solvents like water as the hydrogen bonds in the molecules are equal in strength to the water water interactions which are also hydrogen bonds, thus the molecules are able to penetrate the structure of water leading to salvation // dissolution // hydration i.e. they are polar like the water molecules.
Summary: Properties of some molecular substances.
Property of compound | Molecular substance | ||||||
Sugar (Sucrose) | Naphthalene | Iodine | Rhombic sulphur | Water | Hydrogen sulphide | ||
Solubility in water | Soluble | Insoluble | Insoluble | Insoluble | - | Slightly soluble | |
Molecular mass | 183 | 128 | 186 | 256 | 18 | 34 | |
Melting point (oC) | 186 | 82 | 113 | 114 | 0 | -85 | |
Boiling point (oC) | - | 218 | 183 | 444 | 100 | -60 | |
Electrical conductivity | Solid | Does not | Does not | Does not | Does not | Does not | Does not |
Molten / solution | Does not | Does not | Does not | Does not | Does not | Does not |
Giant Covalent Structures/Giant Atomic Structures.
- Are molecular substances in which atoms are linked throughout the whole structure by very strong covalent bonds from one atom to the next.
- The result is an indefinite number of atoms which are all covalently bonded together.
- This pattern occurs repeatedly throughout the structure leading to a giant of atoms all covalently bonded.
Examples:
Diamond.
- Is an allotrope of carbon.( allotropes are different crystalline forms of the same element in the same physical state.)
- In diamond, each carbon atom is bonded to four other carbon atoms by strong covalent bonds.
- The carbon atoms in diamond are covalently bonded into an octahedral pattern , which repeats itself in all directions resulting into a giant atomic structure.
- Since each carbon atom is bonded to four others, all the four valence electrons in each carbon are used in bonding hence no delocalized electrons in the structure of diamond.
- Diamond is the hardest substance known due to the fact that all the atoms are covalently bonded together and are closely packed together.
Diagram: Structure of diamond:
Properties of diamond.
- Has high melting and boiling points. It has a giant atomic structure with strong covalent bonds throughout the structure which require large amounts of energy to break.
- It is insoluble in water because It is non-polar and thus cannot dissolve in polar water molecules since there are no intermolecular interactions which would facilitate penetration into the water molecules for dissolution to occur.
- Does not conduct heat and electricity. Each carbon atom in the structure of diamond is bonded to four others hence uses all its four valence electrons in bonding and thus lacks any delocalized electrons for electrical conductivity.
- It is the hardest substance known. All carbon atoms are compactly bonded in a continuous octahedral pattern with strong covalent bonds throughout the structure which are very difficult to break.
Graphite.
- Is also an allotrope of carbon.
- In graphite, each carbon atom is bonded to three other carbon atoms by strong covalent bonds.
- Since each carbon atom is bonded to only three others, only three of the four valence electrons in each carbon are used in bonding hence presence delocalized electrons in the structure of graphite.
- This explains the electrical conductivity of graphite.
- The carbon atoms in graphite are covalently bonded into hexagonal layers, which are joined to each other by weak Van der Waals forces.
- The presence of weak Van der Waals forces explains the slippery nature of graphite.
Diagram: Structure of graphite.
Properties of Graphite.
- Have high melting and boiling points, since It has a giant atomic structure with strong covalent bonds throughout the hexagonal layers which require large amounts of energy to break. Even though there are Van der Waals between the layers the effect of the large number of covalent bonds still contribute to high melting and boiling points in graphite.
- Insoluble in water. It is non-polar and thus cannot dissolve in polar water molecules since there are no intermolecular interactions which would facilitate penetration into the water molecules for dissolution to occur.
- It is a good conductor heat and electricity. Each carbon atom in the structure of graphite is bonded to three others hence uses only three of its four valence electrons in bonding. This leads to presence of delocalized electrons in the structure of graphite which conducts heat and electricity.
- It is soft and slippery. The carbon atoms in graphite are covalently bonded into hexagonal layers which are joined to each other by weak Van der Waals forces. The weak Van der Waals forces easily slide over each other when pressed hence the soft and slippery feel.
Silicon (IV) oxide.
- Is a covalent compound of silicon and oxygen.
- Silicon has four electrons in its outermost energy level while oxygen has six.
- Each silicon atom is bonded to four oxygen atoms by strong covalent bonds.
- Each oxygen atom is bonded to two silicon atoms by strong covalent bonds.
- This means there are no delocalized electrons in the structure of silicon (IV) oxide making it unable to conduct electric current.
- The silicon and oxygen atoms are all covalently bonded together (by strong covalent bonds) in a repeated manner leading to a giant of covalent bonds throughout the structure.
- The extra ordinarily strong covalent bonds in silicon (IV) oxide throughout the structure contribute to the very high melting (1728oC) and boiling (2231oC) points.
Diagram: structure of silicon (IV) oxide.
Properties of giant atomic / giant covalent structures.
- Have high melting and boiling points
- They are non-conductors of heat and electricity with the exception of graphite
- They are insoluble in water
- Most are generally very hard, with the exception of graphite
The Metallic Bond.
- Is a bond formed due to electrostatic attraction between the positively charged nuclei and the negatively charged delocalized electrons that hold atoms together.
Formation of a metallic bond.
- In a metal there are usually many atoms surrounding any one atom.
- The valence electrons of any one atom are therefore mutually attracted to many nuclei.
- This leads to a situation in which the positive nuclei appear to be immersed in a sea of mobile electrons.
- The sea of mobile electrons are said to be delocalized which explains the ability of substances with metallic bonds to conduct electric current.
- This pattern of positive nuclei in a sea of electrons is repeated many times throughout the structure leading to a giant metallic structure, the only structure due to metallic bonds.
Diagram: model of the giant metallic structure.
Properties of the giant metallic structure.
- They have high melting and boiling points. They have strong metallic bonds throughout the structure which need large amounts of energy to break. The strength of the metallic bond increases with decrease in atomic size, as well as with increase in the number of delocalized electrons.
- Thus metals with smaller atoms and more delocalized electrons tend to have stronger metallic bonds hence higher melting and boiling points.
- This explains why the melting and boiling points of metallic elements in period three increase from sodium to aluminium. - They are good conductors of heat and electricity. The have delocalized electrons which heat and electric current.
- The electrical conductivity of metals increases with increase in the number of delocalized electrons in each atom in the structure.
- This explains why aluminium metal is a better conductor of heat and electricity than both magnesium and sodium. - They are insoluble in water since there are no dipoles in the giant metallic structure and are thus non-polar, so cannot dissolve in polar water molecules.
Summary: some physical properties of metals
Metal | Valency | Melting point (oC) | Boiling point (oC) | Atomic radii (nm) | Electrical conductivity |
Lithium | 1 | 180 | 1330 | 0.133 | Good |
Sodium | 1 | 98 | 890 | 0.155 | Good |
Potassium | 1 | 64 | 774 | 0.203 | Good |
Magnesium | 2 | 651 | 1110 | 0.136 | Good |
Aluminium | 3 | 1083 | 2582 | 0.125 | Good |
Summary: Comparing Various Types of Structures.
Attribute | Giant metallic | Giant atomic / giant covalent | Giant ionic | Giant metallic |
1. Structure i. Examples |
Na, Fe, Cu. | Diamond, SiC, SiO2 | Ca2+ , O2- ,(K+)2SO42- , Na+ , Cl- , | I2 , S8 , C10H8 , HCl, CH4 . |
ii. Constituent particles |
Atoms | Atoms | Ions | Molecules |
iii. Type of substance compound | Metal element with low electronegativity | Non-metal element in group IV or its compound. | Metal/non-metal compound (a compound of elements with a large difference in electronegativity) | Non-metal element or non-metal/non-metal compound (elements with high electronegativity) |
2. Bonding: In the solid |
Attraction of outer mobile electrons for positive nuclei binds atoms together by strong metallic bonds | Atoms are linked through the whole structure by very strong covalent bonds from one atom to the next. | Attraction of positive ions for negative ions results in strong ionic bonds | Strong covalent bonds hold atoms together within the separate molecules; separate molecules are held together by weak intermolecular forces. |
3. Properties. i. Volatility: State at room temp. |
Non-volatile. Very high melting and very high boiling points. Usually solid | Non-volatile. Very high melting and very high boiling points. Solid | Non-volatile. Very high melting and very high boiling points. Solid | Volatile. Low melting and low boiling points. Usually gases or volatile liquids |
ii. Hardness / malleability | Hard, yet malleable. | Very hard and brittle | Hard and brittle | Soft |
iii. Conductivity | Good conductors when solid or molten. | Non-conductors in any state (except graphite) | Non-conductors when solid; good conductors when molten or in aqueous solution (electrolytes) | Non-conductors when solid, molten and in aqueous solution. (A few like HCl react with water to form electrolytes) |
iv. Solubility | Insoluble in polar and non-polar solvents but soluble in molten metals |
Insoluble in all solvents | Soluble in polar solvents (e.g. H2O), insoluble in polar solvents like tetrachloromethane, CCl4 . | Polar molecules e.g. HCl are soluble inpolar solvents like water; but insoluble in non-polar solvents like CCl4 and vice-versa. |
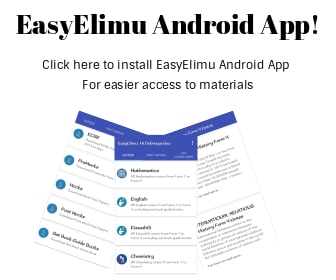
Types of Bonds Across a Period.
- The number of valence electrons play an important role in determination of chemical bonding.
- Across a period in the periodic table, the nature of the bonds varies from metallic to covalent.
- The structure also thus varies from giant metallic to simple molecular.
- Thus similar compounds of the elements in period 3 will also exhibit variation in bond types, structures and properties.
Variation in Bond Types in Oxides of Period Three Elements.
Oxide | Na2O | MgO | Al2O3 | SiO2 | P2O5 | SO2 | Cl2O7 |
Physical state | Solid | Solid | Solid | Solid | Solid | Gas | Gas |
M.P (oC) | 1193 | 3075 | 2045 | 1728 | 563 | -76 | -60 |
B.P (oC) | 1278 | 3601 | 2980 | 2231 | 301 | -10 | -9 |
Structure | Giant ionic | Giant ionic | Giant ionic | Giant atomic | Molecular | Molecular | Molecular |
Bonding | Ionic | Ionic | Ionic | Covalent | Covalent with Van der Waals | Covalent with Van der Waals | Covalent with Van der Waals |
Nature of oxide | Basic (alkaline) | Basic (weakly alkaline) | Amphoteric | Acidic | Acidic | Acidic | Acidic |
Solubility in water | Dissolves to form an alkaline solution | Dissolves to form an alkaline solution | Insoluble | Insoluble | Dissolves in water to form acidic solution. | Dissolves in water to form acidic solution. | Dissolves in water to form acidic solution. |
Reaction with acids | Reacts to form salt and water. | Reacts to form salt and water. | Reacts to form salt and water. | No reaction | No reaction | No reaction | No reaction |
- The melting and boiling points of the magnesium oxide is higher than that of sodium oxide since Both have giant ionic structures. However the electrostatic forces of attraction between magnesium ions and oxide ions are stronger due to the fact that magnesium ion has a charge of +2 and is smaller in size than the sodium ion.
Effect of Bond Type on Properties of Chlorides of Period 3 Elements
- Most period 3 elements form stable chlorides.
- The trend in bond types, structures and properties of chlorides of period 3 elements show variation across the period.
Properties:
Reaction with water.
Procedure:
- A test tube is half filled with water and initial temperature of the water recorded.
- A spatula end full of sodium chloride is added and stirred until it dissolves.
- The highest temperature attained when all the solid dissolves is recorded and the temperature change calculated.
- Two three drops of universal indicator are added and the pH of the solution noted and recorded.
Observations:
Chloride | Observations | ||
Solubility | Temperature change (oC) | pH of solution | |
Sodium chloride | Dissolves | Drop in temperature | 7 |
Magnesium chloride | Dissolves | Slight increase | 6.5 |
Aluminium chloride | Hydrolyzed | Increases | 3 |
Silicon (IV) oxide | Hydrolyzed | Increases | 2 |
Phosphorus (III) chloride | Hydrolyzed | Increases | 2 |
Phosphorus (V) chloride | Hydrolyzed | Increases | 2 |
Explanations.
Sodium and magnesium chlorides.
- Sodium chloride dissolves in water causing a slight drop in temperature.
- Magnesium dissolves readily with a small increase in temperature.
- Both chlorides are ionic and when added to water there is an immediate attraction of polar water molecules for ions in the chlorides.
- The solid thus readily dissolves forming aquated ions such as Na+(aq) and Cl-(aq) .
- These are separate metal and non-metal ions surrounded by polar water molecules.
- Since there is no production of either H+ or OH- ions the solutions are neutral.
Equations:
NaCl(s) + H2O(l) → Na+(aq) + Cl-(aq) + H2O(l) .
MgCl2(s) + H2O(l) → Mg2+ (aq) + 2Cl- (aq) + H2O(l) .
Anhydrous aluminium chloride.
- It exists in molecular form as a dimeric molecule of Al2Cl6 .
- The dimeric molecule is formed when aluminium chloride (AlCl3 ) molecules vapour condense and combine forming larger molecules of Al2Cl6 .
Diagram: Formation of a dimer in aluminium chloride.
- When added to water aluminium chloride is hydrolyzed to form an acidic solution.
- By so doing it behaves like a covalent chloride rather than an ionic chloride.
- The hydrolysis is an exothermic reaction accompanied by release of heat hence the increase in temperature.
Explanations.
- The hydrolysis is due to the very small but highly charged aluminium ion, Al3+ .
- The Al3+ draws electrons away from its surrounding water molecules and causes them to give up H+ ions.
- This reaction usually involves aluminium ions combining with six water molecules to form hexa-aqua-aluminium (III) ions which dissociate to give H+ .
Equation:
[Al(H2O)6]3+(aq) → [Al(H2O)5(OH)]2+(aq) + H+(aq)
- This reaction can be simplified as follows:
- Aluminium chloride reacting with water and hydrolyzing to give HCl(aq) as one of the products.
Equation:
Al2Cl6(s) + 6H2O(l) → 2Al(OH)3(aq) + 6HCl(aq)
- Then the HCl dissociates to give H+ and Cl-
Equation
6HCl(aq) → H+(aq) + Cl-(aq)
- It is the resultant H+ that confer the solution its acidic properties.
Silicon (IV) chloride
- Undergoes hydrolysis in water in an exothermic reaction producing a lot of heat.
- The products of the hydrolysis are silicon (IV) oxide solid and hydrogen chloride gas.
- The hydrogen chloride gas immediately dissolves in the water to form hydrochloric acid.
- The hydrochloric acid dissociated to liberate H+ which leads to acidic conditions.
Equations:
SiCl4(s) + 2H2O(l) → SiO2(s) + 4HCl(aq)
Then: 4HCl(aq) → 4H+(aq) + 4Cl-(aq)
Phosphorus (III) chloride and phosphorus (V) chloride
- Both undergo hydrolysis in water in an exothermic reaction producing a lot of heat.
- The products of the hydrolysis are phosphorus (III) acid and phosphoric (V) acid respectively, and hydrogen chloride gas.
- The hydrogen chloride gas immediately dissolves in the water to form hydrochloric acid.
- The hydrochloric acid dissociated to liberate H+ which leads to acidic conditions.
Equations:
With phosphorus (III) chloride:
PCl3(s) + 2H2O(l) → H3PO3(s) + 3HCl(aq)
Then: 3HCl(aq) → 3H+(aq) + 3Cl-(aq) .
With phosphorus (V) chloride:
PCl5(s) + 4H2O(l) → H3PO4(s) + 5HCl(aq)
Then: 5HCl (aq) → 5H+(aq) + 5Cl-(aq) .
Trends in Bond Types and Properties of Chlorides of Elements in Period 3
Property | Period 3 chloride | |||||
Formula | NaCl | MgCl2 | Al2Cl6 | SiCl4 | PCl3 & PCl5 | SCl2 |
Physical state at RT | Solid | Solid | Solid | Liquid | Liquid | Liquid |
M.P (oC) | 801 | 714 | Sublimes at 180oC | -70 | Sublimes at -94oC | -78 |
B.P (oC) | 1467 | 1437 | - | 57 | - | Decomposes at 57oC |
Conductivity | Good | Good | V. Poor | nil | nil | nil |
Structure | Giant ionic | Giant ionic | Molecular dimer | Molecular | Molecular | Molecular |
Bond type | Ionic | Ionic | Ionic / covalent | covalent | covalent | covalent |
Effect on water | Readily dissolves | Readily dissolves | Hydrolyzed to give HCl | Hydrolyzed to give HCl | Hydrolyzed to give HCl | Hydrolyzed to give HCl |
pH of solution | 7 | 6.5 | 3 | 2 | 2 | 2 |
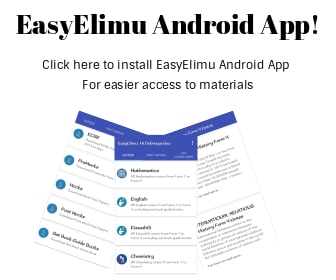
Summary: Characteristics of Bonds
Property | Substances with | ||
Covalent bonds | Ionic bonds | Metallic bonds | |
Electrical conductivity | Non-conductors except graphite | - Solids do not conduct. - Aqueous solutions and molten state conduct |
Conducts |
Thermal conductivity | Non-conductors except graphite | Do not conduct | Conducts |
Melting point (oC) | - Low for molecular substances - High for giant atomic structures |
Usually high | Generally high |
Boiling point (oC) | - Low for molecular substances - High for giant atomic structures |
Usually high | Generally high |
Solubility | Generally insoluble in water but soluble in organic solvents | Generally soluble in water | Some metals react with water |
Download STRUCTURE AND BONDING - Chemistry Notes Form 2.
Tap Here to Download for 50/-
Get on WhatsApp for 50/-
Why download?
- ✔ To read offline at any time.
- ✔ To Print at your convenience
- ✔ Share Easily with Friends / Students